Can this research team reverse climate change?
Global temperatures are increasing; polar ice caps are melting; sea levels are rising: our planet is in jeopardy, and much of this is a result of rising levels of CO2. Cars, planes, food, housing materials, deforestation – our very lifestyles – are resulting in soaring carbon dioxide (CO2) levels that are wreaking havoc on the environment.
We need to reduce CO2 levels and FAST.
WHAT CAN WE DO?
• Trees and plants absorb CO2, so reforestation would be a good place to start
• Changing to renewable energy sources would reduce the amount of fossil fuel combustion and therefore the amount of CO2 released
• Simple lifestyle changes such as walking or cycling instead of taking the car would also help reduce CO2
But reducing the amount of CO2 we produce isn’t enough; we need to remove the CO2 that is already there – a process known as negative emissions. Could a chemical engineer develop a product that could remove the carbon dioxide from the air?
WHAT IS PROFESSOR CHRIS JONES DOING TO TACKLE CLIMATE CHANGE?
Chris is a chemical engineer from the Georgia Institute of Technology, USA. As a chemical engineer, he takes chemicals and raw materials and develops them into useful products for society. In one ambitious project, he is using amine-oxide hybrid materials to create a device that will remove this CO2 from the ambient air, i.e. the air we breathe. This process is called direct air capture (DAC).
Carbon capture technologies that are able to ‘capture’ CO2 already exist, but these tend to reduce CO2 emissions locally. Capturing carbon within the flue of a power station is an example of a localised carbon capture technology. DAC, on the other hand, is a type of negative emissions technology (NET). This means that it extracts CO2 that has been released from a multitude of sources over a long period of time, including from cars and aeroplanes as well as power plants and factories.
Negative emissions technology is therefore able to reduce the amount of CO2 in the air and has the potential to reverse climate change!
HOW DOES CHRIS’ DAC TECHNOLOGY WORK, EXACTLY?
In order to remove the dilute amounts of CO2 in the air, a material has to be able to bind to the gas very strongly. Chris’ amine-oxide material binds to CO2 through acid-base interactions. You’ll have learnt about acids and bases at school: acids, such as citric acid found in lemons, have a low pH; whilst bases, such as sodium hydroxide found in cleaning products, have a high pH. Alkali is a term for bases that are soluble in water.
CO2 is weakly acidic and the amine groups (-NH2) are basic. When CO2 passes by the amine groups, an interaction occurs, and the carbon is caught or ‘captured’ by the amines (see “What’s the chemistry?”).
To engineer this chemical reaction into a useful tool, Chris’ group used an oxide support, such as aluminium oxide, built into a monolithic, honeycomb structure, which has a large surface area. The amines were introduced in long chains or polymers with many side chains, and were attached to this honeycomb.
The next development was to incorporate an air-blowing machine (imagine a leaf blower) into the device. This blows large quantities of air through the honeycomb structure. As the air passes through, the amine materials ‘grab’ the CO2, removing it from the air. The remaining air blows straight out the other end!
Chemical engineers often work closely with industry, and Chris is no exception. He is collaborating with a start-up company called Global Thermostat. “Global Thermostat believe their technology, if successfully deployed at scale, could act as a global temperature controller for the Earth” he says. “Take out more CO2 to cool; slow CO2 removal to warm!”
WHAT HAPPENS TO THE CARBON?
When the amine-oxide material is sufficiently loaded with CO2, the device is heated up, which causes the gas to ‘fall off’ the material. The resulting concentrated CO2 product must then be stored – usually by compressing it to high pressure and depositing it underground – hopefully for hundreds of years. Alternatively, it can be sold as a commodity: CO2 has various industrial uses, from food to oil and chemicals. However, to be classed as a negative emissions technology, the carbon must be packed away somewhere so that it can’t escape back into the atmosphere.
Reference
https://doi.org/10.33424/FUTURUM17
WHAT IS PROFESSOR CHRIS JONES DOING TO TACKLE CLIMATE CHANGE?
Chris is a chemical engineer from the Georgia Institute of Technology, USA. As a chemical engineer, he takes chemicals and raw materials and develops them into useful products for society. In one ambitious project, he is using amine-oxide hybrid materials to create a device that will remove this CO2 from the ambient air, i.e. the air we breathe. This process is called direct air capture (DAC).
Carbon capture technologies that are able to ‘capture’ CO2 already exist, but these tend to reduce CO2 emissions locally. Capturing carbon within the flue of a power station is an example of a localised carbon capture technology. DAC, on the other hand, is a type of negative emissions technology (NET). This means that it extracts CO2 that has been released from a multitude of sources over a long period of time, including from cars and aeroplanes as well as power plants and factories.
Negative emissions technology is therefore able to reduce the amount of CO2 in the air and has the potential to reverse climate change!
HOW DOES CHRIS’ DAC TECHNOLOGY WORK, EXACTLY?
In order to remove the dilute amounts of CO2 in the air, a material has to be able to bind to the gas very strongly. Chris’ amine-oxide material binds to CO2 through acid-base interactions. You’ll have learnt about acids and bases at school: acids, such as citric acid found in lemons, have a low pH; whilst bases, such as sodium hydroxide found in cleaning products, have a high pH. Alkali is a term for bases that are soluble in water.
CO2 is weakly acidic and the amine groups (-NH2) are basic. When CO2 passes by the amine groups, an interaction occurs, and the carbon is caught or ‘captured’ by the amines (see “What’s the chemistry?”).
To engineer this chemical reaction into a useful tool, Chris’ group used an oxide support, such as aluminium oxide, built into a monolithic, honeycomb structure, which has a large surface area. The amines were introduced in long chains or polymers with many side chains, and were attached to this honeycomb.
The next development was to incorporate an air-blowing machine (imagine a leaf blower) into the device. This blows large quantities of air through the honeycomb structure. As the air passes through, the amine materials ‘grab’ the CO2, removing it from the air. The remaining air blows straight out the other end!
Chemical engineers often work closely with industry, and Chris is no exception. He is collaborating with a start-up company called Global Thermostat. “Global Thermostat believe their technology, if successfully deployed at scale, could act as a global temperature controller for the Earth” he says. “Take out more CO2 to cool; slow CO2 removal to warm!”
WHAT HAPPENS TO THE CARBON?
When the amine-oxide material is sufficiently loaded with CO2, the device is heated up, which causes the gas to ‘fall off’ the material. The resulting concentrated CO2 product must then be stored – usually by compressing it to high pressure and depositing it underground – hopefully for hundreds of years. Alternatively, it can be sold as a commodity: CO2 has various industrial uses, from food to oil and chemicals. However, to be classed as a negative emissions technology, the carbon must be packed away somewhere so that it can’t escape back into the atmosphere.
“Until recently, there hasn’t been a strong market for NETs and DAC,” says Chris. “And therefore fewer ways for companies to monetise DAC technologies. While the amine-oxide hybrid materials we work with are relatively easy to make, they’re not being produced at commercial scale yet. As the market develops, I anticipate an array of companies, both established and start-ups, will be poised to manufacture such materials. We’re working with several of them today.”
The first commercial DAC unit on the market was produced by a company called Climeworks and is in use in Switzerland. Hopefully, this is the start of many.
However, while large scale use of DAC could negate the effects of our fossil fuel-burning lifestyles, it’s vital that we move to renewable and more sustainable energy sources. What’s exciting is that Chris’ technology could help give us more time: “This would allow a slower transition to a renewable, energy-rich future, offering less economic disruption and loss of jobs associated with fossil energy,” he says. “It could be both a climate and market stabiliser.”
Let’s describe acids and bases even more scientifically. There are three theories of acids and bases: the Bronsted-Lowry, Arrhenius and Lewis theories.
According to the Bronsted-Lowry definition, an acid is a proton donor that can donate a positive ion, usually a hydrogen ion (H+). A base is a proton acceptor that can receive a positive ion, again, usually H+. These charge differences enable the acid and base to interact to make a neutral product.
The Arrhenius theory describes acids as substances that produce hydrogen ions in solution, and bases as substances that produce hydroxide ions in solution. When the hydrogen and hydroxide ions interact, they produce water, a neutral product.
Finally, Lewis theory gives the broadest definition, describing acids as electron pair acceptors and bases as electron pair donors.
In Chris’ device, the acid is carbon dioxide and the base is the amine group. CO2 + 2 R-NH2 —> R-NH3+ + R-NHCOO– , where R represents a hydrocarbon chain.
When carbon dioxide and amine groups interact, a chemical reaction takes place, creating strong bonds. The equation above shows the formation of an alkylammonium carbamate, which is the key product formed from reactions of a solid-supported amine and CO2. The amines act as bases, with one amine donating electrons (Lewis theory) to the acidic molecule CO2, forming a covalent N-C bond. A second amine group removes a hydrogen ion from this product, producing an ammonium R-NH3+ group. The final product is a cation-anion pair, the alkylammonium carbamate.
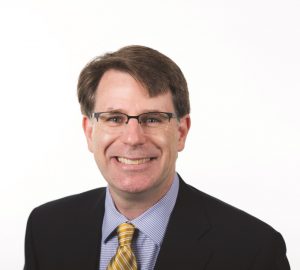
PROF CHRIS JONES
Professor of Chemical and Biomolecular Engineering
Georgia Institute of Technology, USA
FIELD OF RESEARCH: Chemical and biomolecular engineering. Specialty: catalysis and carbon capture field of research
RESEARCH PROJECT: Chris’ research group is developing materials that extract carbon dioxide directly from the air in a process called ‘direct air capture’. This is one of the few methods that could reverse climate change.
FUNDERS: US Federal Government: National Science Foundation, Department of Energy; Industry: The Dow Chemical Company, Global Thermostat LLC
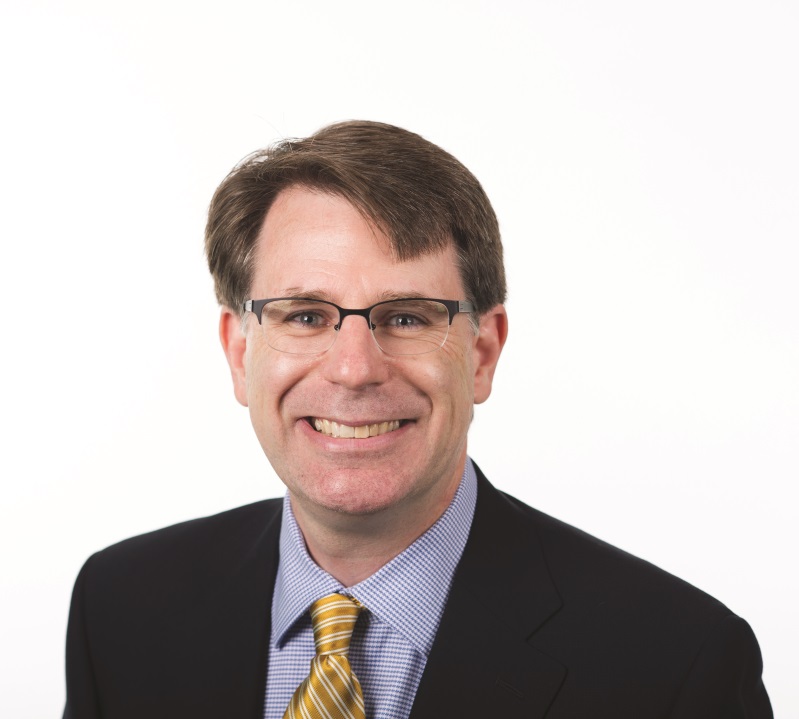
PROF CHRIS JONES
Professor of Chemical and Biomolecular Engineering
Georgia Institute of Technology, USA
FIELD OF RESEARCH: Chemical and biomolecular engineering. Specialty: catalysis and carbon capture field of research
RESEARCH PROJECT: Chris’ research group is developing materials that extract carbon dioxide directly from the air in a process called ‘direct air capture’. This is one of the few methods that could reverse climate change.
FUNDERS: US Federal Government: National Science Foundation, Department of Energy; Industry: The Dow Chemical Company, Global Thermostat LLC
If you enjoy solving problems, perhaps chemical engineering is for you. Using knowledge of science, computing and maths, chemical engineers turn raw materials into useful products, overcome hurdles and develop new methods. They bridge the gap between research and manufacturing.
Chemical engineers design, modify and improve the way we produce a vast array of everyday goods: face creams, chocolate, fuels, medicines, sustainable energy, waste treatment, the development of stem cell therapies. Wherever your interest lies, there is a niche for you.
Chemical engineers often work in large teams of people from various backgrounds such as mechanical, electrical and other types of engineers, as well as with scientists, business professionals and policymakers. “Chemical engineering is a powerful field that can open doors to a variety of careers,” says Chris.
Careers in chemical engineering require an undergraduate degree, either a bachelor’s degree (BEng), which takes 3-4 years and gives a solid understanding of the principles of chemical engineering, or a Masters degree (MEng), which typically lasts a year longer, providing a more extensive knowledge base, usually in a specific area of chemical engineering. Employers look for candidates with relevant work experience, so when applying for university courses, it’s sensible to select ones with an internship or industrial placement scheme.
During their early career, many chemical engineers aim to achieve chartered status – a qualification that demonstrates experience and expertise.
Graduates can work a wide range of industries, including:
- Food and beverages • Biotechnology • Consumer products (detergents, batteries, automobile, etc.) • Energy – oil / gas as well as renewable • Polymers / plastics • Chemicals • Business management and consulting • Pharmaceuticals • Water • Numerous graduate schools (medical, dentistry, pharmacy, law, business, etc.).
Graduate starting salaries for chemical engineers are high and are in the region of $68,520 in the US. The average salary for a chemical engineer is $112,430, although this varies according to location and sector.
Teenlife promote various engineering summer schools in the US – check them out!
WHY DID YOU CHOOSE TO STUDY CHEMICAL ENGINEERING?
Through middle school, I had little knowledge of what types of careers were available beyond the obvious ones (doctor, lawyer, teacher, police, etc.), but I knew I liked science, based on success in science subjects and positive feedback from teachers. In high school, I took my first chemistry course and found it was easy to learn, so I took additional chemistry courses. My chemistry teacher encouraged me further, but also mentioned that if I was good at maths, I should consider being a chemical engineer because he believed there were more job opportunities for a BS chemical engineer than a BS chemist. I took both chemistry and chemical engineering courses in my first two years of university and found I really liked chemical engineering, so decided to take that route. I chose this major primarily because I felt I was good at it and therefore enjoyed it, more so than with any specific career path in mind.
IS THERE ANYTHING THAT YOU WOULD DO DIFFERENTLY IF YOU COULD GO BACK IN TIME TO YOUR PRIMARY AND SECONDARY SCHOOL YEARS?
Yes, I would study a foreign language at an early enough age to become fluent. Because English is the language of science and the primary international language, relatively few young people from English-speaking countries become fluent in a second language. Do it! It opens doors culturally, and the earlier you start, the easier it is to become fluent.
ARE THERE ANY STEREOTYPES ABOUT SCIENTISTS AND ENGINEERS THAT YOU WISH TO BREAK?
Many people envision scientists and engineers as people who work in isolation, in a lab and away from people. In reality, science has given me a great balance between social activities and alone time. I love studying a subject deeply on my own. When I was younger, I loved working on my own project in the lab alone. But the global research community in carbon capture and catalysis, my two fields, is well-connected and close-knit. Through international research conferences, I’ve developed close friends in 40+ countries around the world. Because scientists and engineers all speak a common language (the scientific method as applied to their specialty subject area), cultural barriers are easily broken down, and friendships are readily made, allowing for a strong social component to the job.
ARE YOU AN ECO-WARRIOR AT HOME AS WELL AS AT WORK?
No, not as much as I should be, and this is a source of constant internal struggle for me. I feel strongly about climate change, conservation and energy efficiency, but I also travel a lot for my job (I fly 50-75k miles per year), which has a huge carbon footprint. I also like to drive my car on racetracks. I grew up in Detroit, the ‘Motor City’, so automobiles are in my blood. Racing is not often viewed as a model activity for energy conservation. But these activities bring me joy, so my research is focused on developing technologies that will allow my travel and racing to be eco-friendly. Check out Formula E racing!
WHAT DO YOU LIKE TO DO IN YOUR FREE TIME?
I love my job, my research, and interacting with my students, so I spend a lot of my free time at work. But I also love to travel (40+ countries to date, and all five major continents covered) and I watch a lot of ice hockey (Detroit Red Wings) and auto racing (Formula 1, Formula E).
Many STEM students think that only maths and science subjects are important for their career. Although these are clearly vital, other skills are also important to be a successful chemical engineer:
- Communication skills are what differentiates a good engineer from a great engineer. The most successful chemical engineers have excellent language skills and are effective communicators
- It is important to be well-rounded. Engineering is about applying your skills to improve humanity, so being comfortable with social sciences like public policy, economics and sociology is helpful
- Trust your gut and choose subject areas that you enjoy
- Be business savvy – many chemical engineers either work in industry, have collaborations with industry, or create start-ups