Why gravitational waves are of supermassive importance
Dr Paul Lasky, Senior Lecturer in the School of Physics and Astronomy at Monash University in Australia, tells us why the recent detection of gravitational waves from outer space has had such a huge impact on his research and science as a whole
Gravitational waves are tiny ripples in the fabric of space-time, which travel at the speed of light. Their existence was first proposed by Henri Poincaré in 1905 and then predicted by Albert Einstein in 1916, as a consequence of his general theory of relativity. However, in the 100 years since then, there was only indirect observational evidence for their existence, which came in the late 1980s. That was until February 2016, when the LIGO Scientific Collaboration announced the first detection of tiny ripples of space-time.
It is difficult to overstate just how important this discovery is; the detection of gravitational waves has opened a new window on the universe. Prior to this, scientists studied the universe through light waves. This includes radio waves, visible light, X rays and gamma rays. Every time an astronomer started looking at the universe through one of these lenses, new discoveries were made. Gravitational waves are no different.
Dr Paul Lasky, Senior Lecturer at the School of Physics and Astronomy, Monash University, is a member of LIGO and is interested in a number of topics under the broad heading of gravitational astrophysics (although he has a particular penchant for gravitational-wave astrophysics). The detection of gravitational waves has had an enormous impact on his research that is still felt to this day.
HOW WERE GRAVITATIONAL WAVES DETECTED?
Imagine two fixed points in space: As a gravitational wave passes between them, the physical distance between them changes – it grows and shrinks in oscillations. If you think of two perpendicular directions, while one direction grows, the other shrinks. Half a gravitational-wave cycle later, the opposites true. The LIGO experiments exploit this fact to measure the difference in time it takes a pulse of a laser to travel in two directions perpendicular to one another.
“The LIGO experiment has four-kilometre long arms. The gravitational wave that we detected on September 14, 2015, caused those four-kilometre arms to change length by about one one-thousandth the size of a proton,” explains Paul. “That is what LIGO detected with one of its experiments. Of course, many different things can cause such an instrument to wobble by such an amount. This is why two identical experiments were built; one in Livingston, Louisiana, and the other in Hanford, Washington (both in the US).”
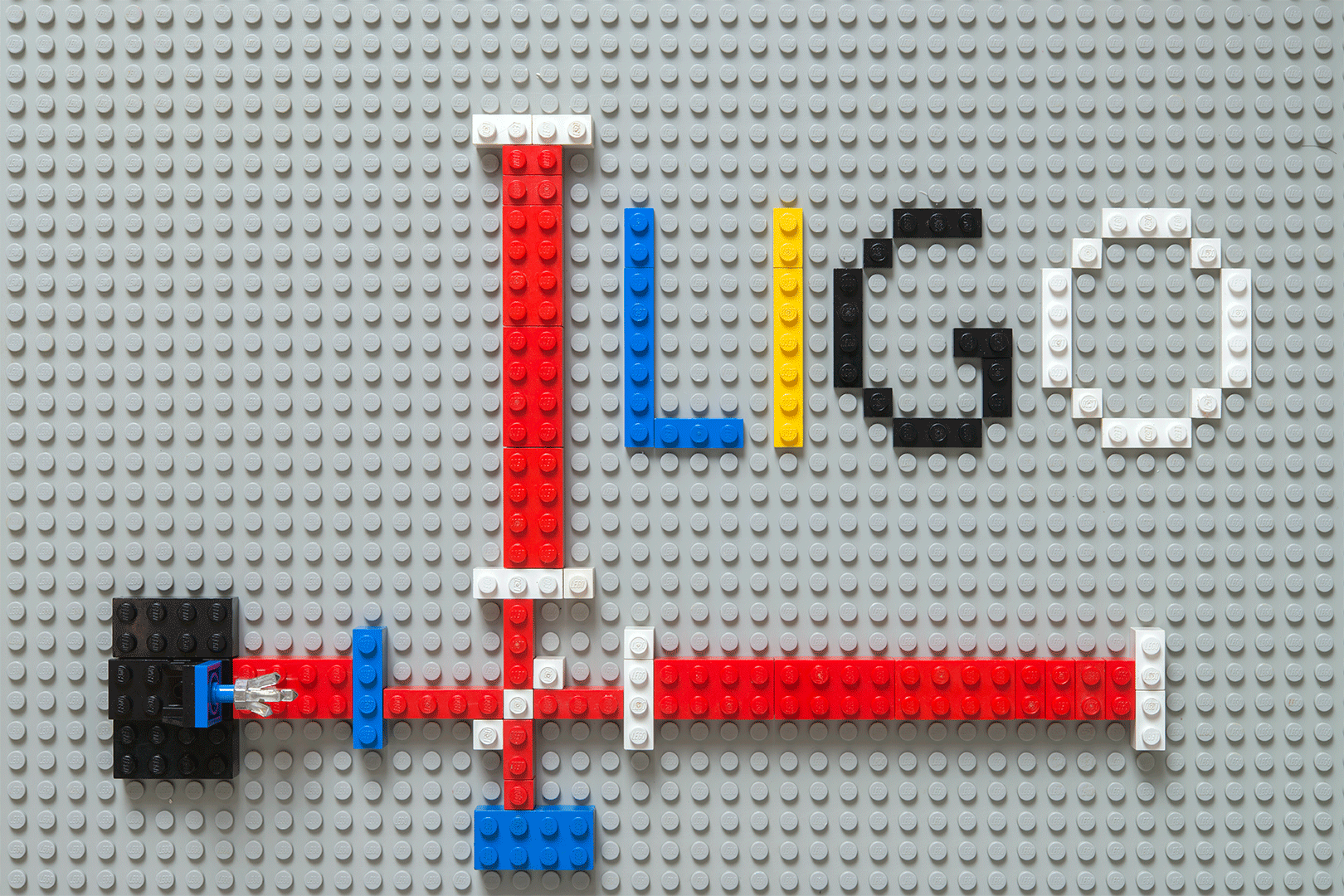
The LIGO experiment
The same signal was detected in both detectors with a difference in arrival time of just seven milliseconds. That difference is explained by the time it took the gravitational waves to pass from one detector to the next; they are separated by ~1,000 km.
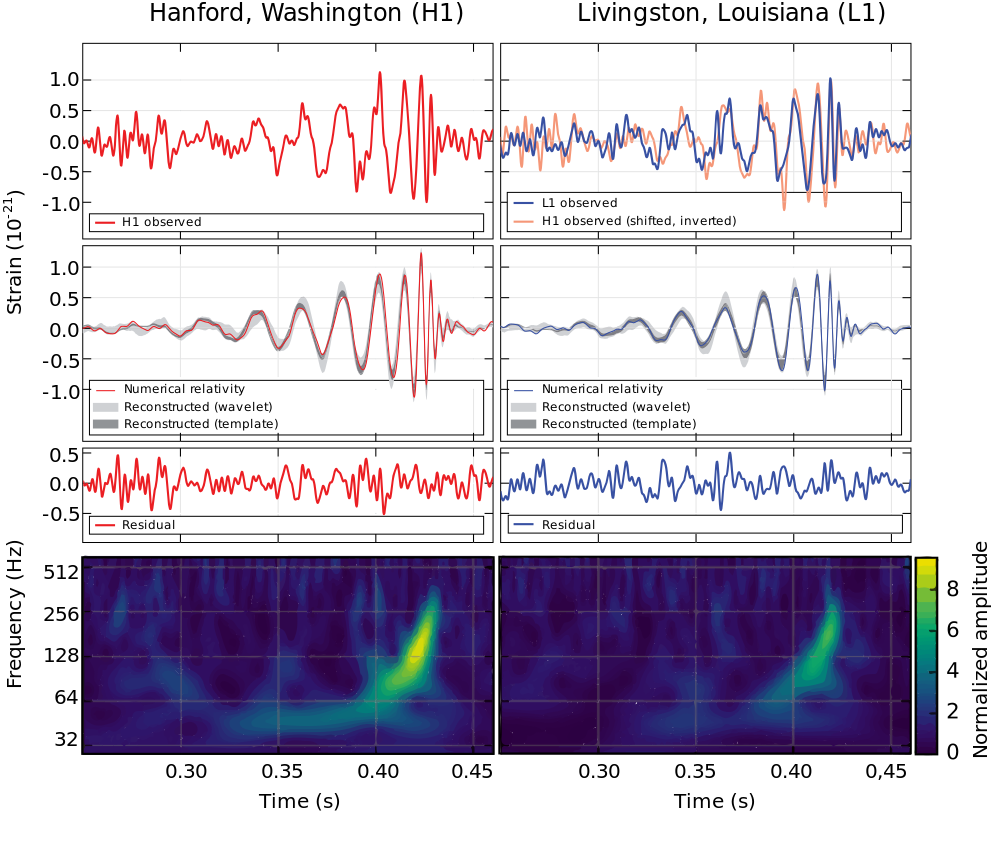
This image shows the gravitational wave signals received by the LIGO instruments at Hanford, Washington (left) and Livingston, Louisiana (right), and comparisons of these signals to the signals expected due to a black hole merger event.
Reference
https://doi.org/10.33424/FUTURUM24
Paul and Greg Ashton (Associate Lecturer from the School of Physics and Astronomy at Monash University) stand in front of an artist’s rendition of the interiors of neutron stars. Photo credit: Carl Knox, Swinburne University/OzGrav
An artist’s impression of merging black holes
Paul deriving some equations on the collapse of stars that form black holes
Artist’s impression of gravitational waves emanating from two neutron stars
Gravitational waves are tiny ripples in the fabric of space-time, which travel at the speed of light. Their existence was first proposed by Henri Poincaré in 1905 and then predicted by Albert Einstein in 1916, as a consequence of his general theory of relativity. However, in the 100 years since then, there was only indirect observational evidence for their existence, which came in the late 1980s. That was until February 2016, when the LIGO Scientific Collaboration announced the first detection of tiny ripples of space-time.
It is difficult to overstate just how important this discovery is; the detection of gravitational waves has opened a new window on the universe. Prior to this, scientists studied the universe through light waves. This includes radio waves, visible light, X rays and gamma rays. Every time an astronomer started looking at the universe through one of these lenses, new discoveries were made. Gravitational waves are no different.
Dr Paul Lasky, Senior Lecturer at the School of Physics and Astronomy, Monash University, is a member of LIGO and is interested in a number of topics under the broad heading of gravitational astrophysics (although he has a particular penchant for gravitational-wave astrophysics). The detection of gravitational waves has had an enormous impact on his research that is still felt to this day.
HOW WERE GRAVITATIONAL WAVES DETECTED?
Imagine two fixed points in space: As a gravitational wave passes between them, the physical distance between them changes – it grows and shrinks in oscillations. If you think of two perpendicular directions, while one direction grows, the other shrinks. Half a gravitational-wave cycle later, the opposites true. The LIGO experiments exploit this fact to measure the difference in time it takes a pulse of a laser to travel in two directions perpendicular to one another.
“The LIGO experiment has four-kilometre long arms. The gravitational wave that we detected on September 14, 2015, caused those four-kilometre arms to change length by about one one-thousandth the size of a proton,” explains Paul. “That is what LIGO detected with one of its experiments. Of course, many different things can cause such an instrument to wobble by such an amount. This is why two identical experiments were built; one in Livingston, Louisiana, and the other in Hanford, Washington (both in the US).”
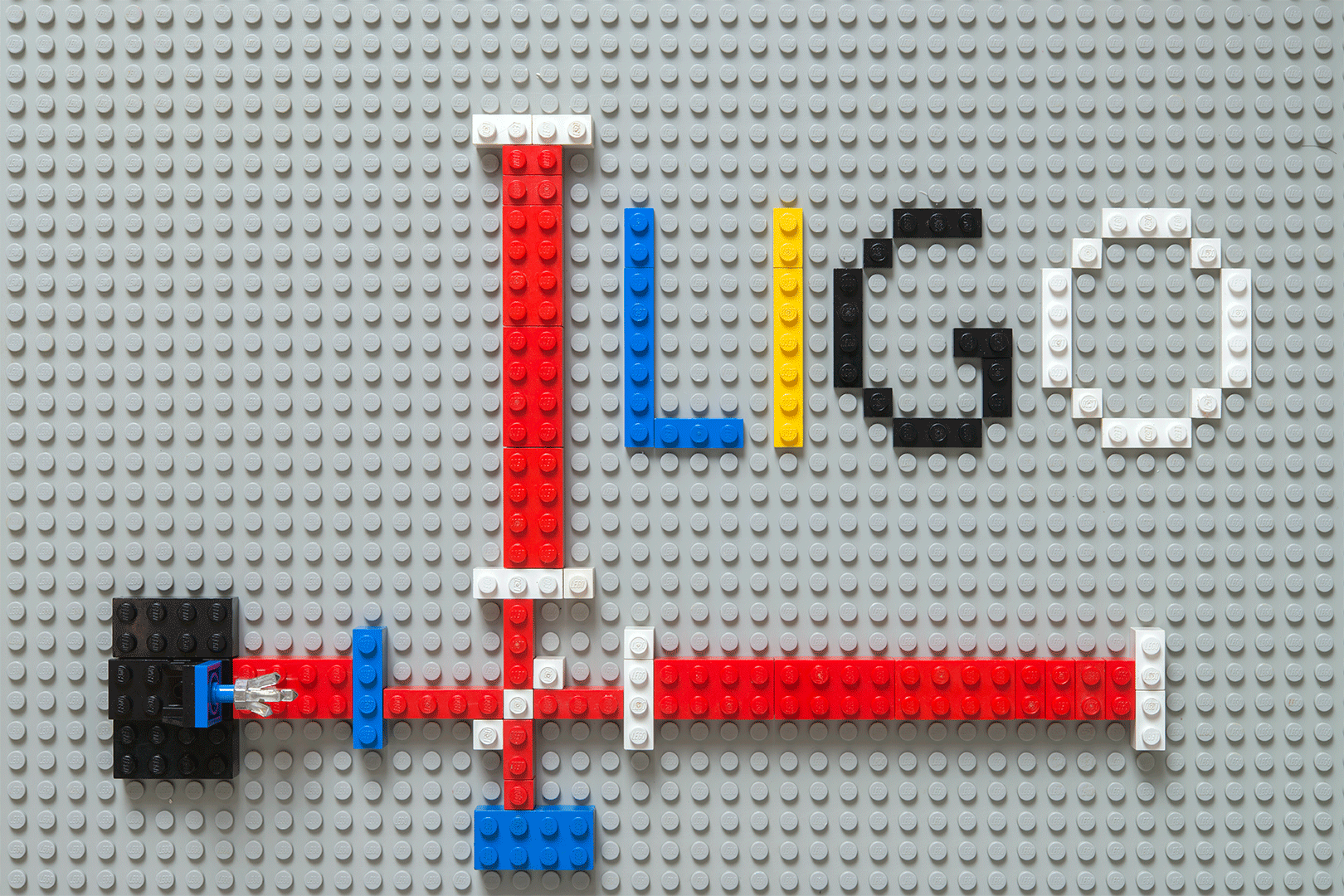
The LIGO experiment
The same signal was detected in both detectors with a difference in arrival time of just seven milliseconds. That difference is explained by the time it took the gravitational waves to pass from one detector to the next; they are separated by ~1,000 km.
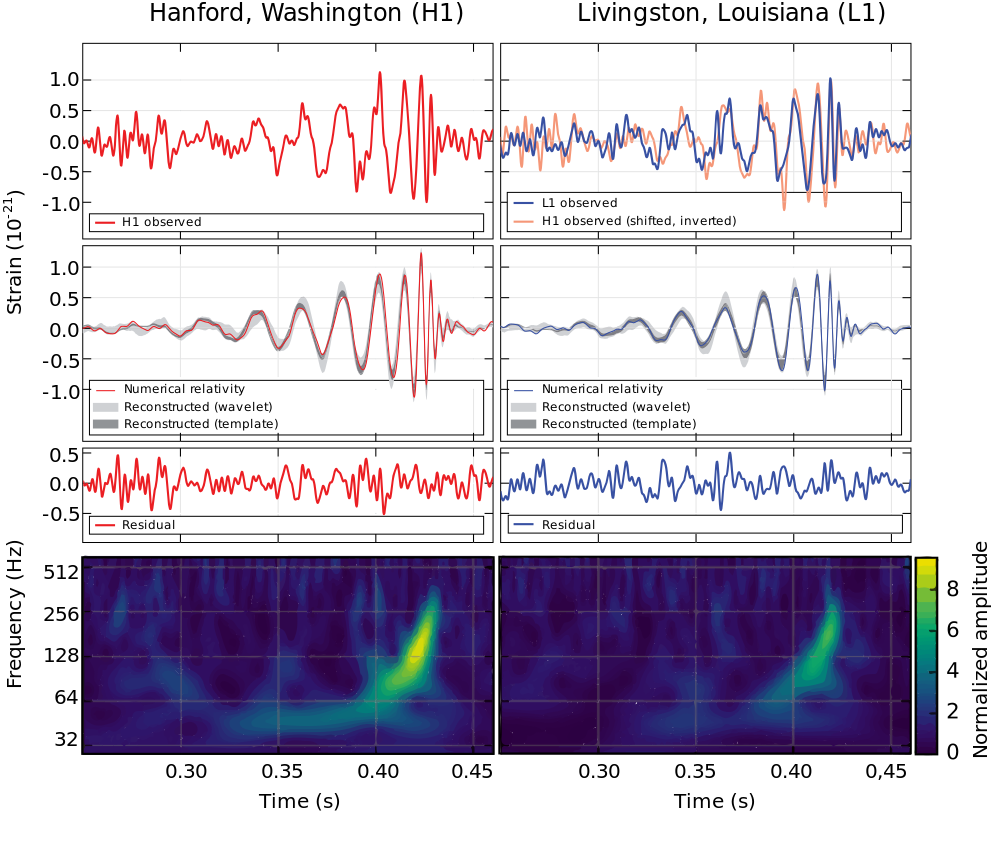
This image shows the gravitational wave signals received by the LIGO instruments at Hanford, Washington (left) and Livingston, Louisiana (right), and comparisons of these signals to the signals expected due to a black hole merger event.
WHAT DOES THIS DISCOVERY MEAN FOR ASTROPHYSICS, OUR PLANET AND OUR SOCIETY?
As well as helping to revolutionise our understanding of the universe, the discovery has enabled scientists to learn new things about phenomena such as black holes, extreme gravity, neutron stars, cosmology, gamma-ray bursts and stellar evolution. “While we’ve already learnt so much in just under four years of discovery, I think the future is even more exciting,” says Paul. “We have spent years predicting different types of sources of gravitational waves that we hope to measure in the coming years and decades, but the most exciting discovery will be the one no one expects.”
WHAT MIGHT WE LEARN ABOUT BLACK HOLES?
Paul is also a member of the Parkes Pulsar Timing Array, and this involves conducting research into the pulsar timing band. A Pulsar Timing Array (PTA) searches for signals on extremely long timescales. While the LIGO detectors can measure gravitational waves from colliding black holes that have similar masses to that of our Sun, those signals are only in the detector for between fractions of a second up to a few seconds. PTAs search for signals on much longer timescales, which correspond to bigger (supermassive) black hole collisions.
Paul and his team are searching for these so- called supermassive black holes, which can be more than a billion times the mass of our Sun. Supermassive black holes are believed to reside in the cores of galaxies. The Event Horizon Telescope recently imaged a black hole in M87 – a 6.6 billion solar mass supermassive black hole in a nearby galaxy. “We know galaxies collide with one another, and we therefore expect the supermassive black holes to find one another, and eventually merge,” explains Paul. “PTAs search for gravitational waves coming from the mergers of such supermassive black holes throughout the universe. At present, we have not seen anything, but the community is hopeful a positive detection is just around the corner.”
The scientific community had to wait one hundred years for the first detection of gravitational waves – and that had a tremendous impact on our understanding of the universe – so waiting for the detection of gravitational waves from supermassive black holes shouldn’t be a problem. Some things are just worth waiting for!
DR PAUL LASKY
ARC Future Fellow and Senior Lecturer, Monash Centre for Astrophysics, School of Physics and Astronomy, Monash University, Australia
FIELD OF RESEARCH: Gravitational Astrophysics
RESEARCH PROJECT: Paul’s research extends across a number of topics that can be filed under the broad heading of gravitational astrophysics. However, he has a particular penchant for gravitational-wave astrophysics.
FUNDERS: Australian Research Council (ARC), OzGrav: ARC Centre of Excellence for Gravitational Wave Discovery
DR PAUL LASKY
ARC Future Fellow and Senior Lecturer, Monash Centre for Astrophysics, School of Physics and Astronomy, Monash University, Australia
FIELD OF RESEARCH: Gravitational Astrophysics
RESEARCH PROJECT: Paul’s research extends across a number of topics that can be filed under the broad heading of gravitational astrophysics. However, he has a particular penchant for gravitational-wave astrophysics.
FUNDERS: Australian Research Council (ARC), OzGrav: ARC Centre of Excellence for Gravitational Wave Discovery
WHAT IS GRAVITATIONAL-WAVE ASTRONOMY?
Gravitational-wave astronomy is a branch of gravitational astrophysics and observational astronomy, more broadly. Its aim is to collect information about objects in space that are an incomprehensible distance away from Earth. Because there is no possibility of ever travelling to the majority of objects being studied, researchers have to use the tools at their disposal, which have often been limited in scope.
The existence of gravitational waves – ripples in space-time – were predicted by Albert Einstein more than 100 years ago. In many ways, confirming the existence of them was seen as something of a “holy grail” for gravitational astrophysicists, not least because of the new opportunities it would afford researchers.
WHY ARE GRAVITATIONAL WAVES SO IMPORTANT TO GRAVITATIONAL ASTROPHYSICS?
Gravitational waves have two properties that are unique and of huge importance to researchers. First, there doesn’t need to be any matter nearby for the waves to be generated by a binary system of uncharged black holes (which would emit no electromagnetic radiation). Second, gravitational waves can pass through any intervening matter without being significantly scattered.
If, for example, somebody is attempting to observe light from a distant star, this could be blocked out by interstellar dust, but gravitational waves will pass right through. Together, these two properties enable gravitational waves to carry information about astronomical phenomena that was previously unattainable.
ARE THERE ANY OPPORTUNITIES FOR CITIZEN SCIENCE WITHIN GRAVITATIONAL ASTROPHYSICS?
Yes. There is a citizen science opportunity called Gravity Spy, which is hosted by the Center for Interdisciplinary Exploration and Research in Astrophysics (CIERA), at Northwestern University in Illinois, USA.
The idea behind the initiative is to pair citizen science with machine learning to help discover gravitational waves. In addition to characterising and eliminating the problem of noise (known as glitches) in detecting gravitational waves, Gravity Spy also promotes gravitational-wave sciences and involves the general public in the scientific progress made.
WHY SHOULD YOUNG PEOPLE TAKE AN INTEREST IN GRAVITATIONAL WAVES?
Gravitational waves are providing a completely new avenue for looking at and understanding the universe around us. Only a handful of times in the history of modern science have completely new ways to study the universe been unearthed. In just four years since the first detection, this field has already started to revolutionise
OPPORTUNITIES IN ASTRONOMY
• According to PayScale, an average salary for an astrophysicist in Australia is $91,000.
• However, Australia’s Science Channel points out that the space industry “generates a lot of employment for engineers, mathematicians, physicists, chemists, biologists, doctors – even lawyers who are devising a regulatory regime covering all of our activities in space”.
• Finding work experience in astronomy is one of the best ways of discovering whether a career in this field is for you. Many institutes in Australia offer work experience placements for high school students. Simply type in “astronomy work experience” in a search engine and you’ll be presented with a long list!
• The Astronomical Society of Australia and Australian Space Agency have lots of resources to help you further your interest in space, including links to local amateur astronomical societies, fact sheets and summer vacation scholarships for university students.
ASK DR PAUL LASKY
WHO OR WHAT DREW YOU TO ASTROPHYSICS IN THE FIRST PLACE?
There have been a number of influences for me. When I was a teenager, my dad read popular science books from people like Kip Thorne, Stephen Hawking, Paul Davies, etc., and I started following his lead. My dad is not a scientist, but he has one of the best analytic brains I have come across and he was able to digest the content of these books with relative ease. This is something that always impressed me; I don’t have my father’s mind, but this didn’t stop me being absolutely fascinated by everything I read in those books.
At high school I had a couple of science teachers that really inspired me. I remember one chemistry lesson in the final years of high school – our teacher walked in, threw the standard curriculum on the floor, and decided to teach us all about quantum mechanics. I was awestruck about the non-deterministic nature of the universe. Probably more importantly, from reading popular science books, I became fascinated by the fact that giants in the field, including people like Einstein, were also unable to comprehend these aspects of the universe. There was – and still is – so much left to discover.
WHAT IS IT ABOUT YOUR RESEARCH THAT FASCINATES YOU?
I love uncovering new things about the universe. I love knowing that, for short periods of time at least, you’re one of the only people on Earth who understands a certain phenomenon or a certain fact about the universe. I think any field of science would give people this thrill.
In terms of astrophysics research, I love furthering my understanding of extreme aspects of the universe. I cannot fathom the size, mass, density and temperature of any of the objects that I study, but that doesn’t mean I can’t understand the way they work! It also doesn’t mean I can’t make predictions for what we should see when we observe them with telescopes or gravitational-wave detectors, and then I actually get a chance to test those predictions and see if we, as a community, were right.
I love my job in general. I still have to pinch myself that I get paid to pursue my hobby. If I don’t find a topic interesting, I don’t work on it. I get to work with incredibly bright and motivated young people who have the world at their feet. I get to mentor them and help them pursue their dream of making contributions at the bleeding edge of science.
WOULD YOU LIKE TO TRAVEL IN SPACE?
Absolutely. I’m fascinated by how gravity works in general and would be amazed to understand the way it affects our daily lives in ways that we don’t even realise. Going into space would give me another appreciation of this.
WHAT ADVICE DO YOU HAVE FOR YOUNG PEOPLE WHO ARE UNCERTAIN ABOUT WHAT TO DO WITH THEIR LIVES?
Follow your passion. I never knew that I wanted a career in astronomy or physics. I always loved learning about the universe but didn’t realise it was something that I would be capable of doing as a long-term career. It was only through following my interest and passion that I was able to forge a career in this field, and now I rarely feel like I’m working. Instead, I truly feel like I’m getting paid to pursue my hobby.
Many people ask me why they should study physics or astronomy and what the job prospects are, but I think this is the wrong question. If you work hard, the jobs will come; passionate people with a solid scientific education are eminently employable in a whole range of fields. You’ll never have trouble finding an excellent, well-paid job with a good science degree. But you’ll also never get a better opportunity to scratch that itch and learn the fundamentals of the universe and its inner workings.
PAUL’S TOP TIPS
1 If you are interested in the field of gravitational astrophysics, you should get a good grounding in both physics and mathematics. However, there are other ways of getting into the field, such as with computer science or electrical engineering backgrounds; these can prove extremely valuable for creating software and mathematical/data-analysis techniques for understanding the complicated data.
2 Attaining a degree is not necessarily the only route into the field. If you have an interest in getting involved in the outreach and science communication side of the field, then there are opportunities out there.
3 Do not study physics or astronomy if the only thing you want is a job at the end of it. You should study these subjects because you are passionate about understanding how the universe works; we are only ever limited by our current understanding of the way things are, so contributing to the development of new ways of looking at the universe can shape the future.